Background
Île-de-France represents 21% of health activities in France with various players such as research centres, universities, hospital services, small and large companies and investors. The richness of the ecosystem of Île-de-France around synthetic biology and biotherapy/bioproduction lies at the heart of this unifying project to promote scientific unity and a common direction.
Today, and despite the existence of many scientific and economic signals demonstrating the importance of biofoundery for biotechnology, there is no such project in France: that’s why the DIM BioConvergence for Health initiative was established. The structuring objective of the DIM is to create a network of distributed biofounderies (with the participation of Sorbonne University, INRAe, Institut Curie, Genopole and Université Paris Cité) to make technologies for life design and engineering accessible and thus position the Île-de-France Region as a key player in biotechnology, bioproduction and biotherapy. This structuring will involve the acquisition and pooling of high-tech equipment dedicated to the molecular engineering of living systems and bioproduction. This will allow a large community to have access to state-of-the-art tools in synthetic biology, bioproduction tools including bioreactors for scaling up. This will make it possible to create genetic circuits, synthetic cells and bioprocesses ondemand.
Another flagship initiative is the CollectivE Digital Intelligence Platform (DICE), led by UMR 1284 within the Learning Planet Institute and Université Paris Cité, which will be set up with a set of digital resources to design, document and bring together BioConvS research projects in a virtual collaborative research space. This platform, open to the general public, will strengthen the capacity for exchange between DIM’s partners and society.
This structuring will also involve the financing of collaborative projects, theses, engineering and post-doctoral jobs between DIM teams, the organization of workshops and conferences and support for the participation of student teams in the “International Genetically Machine Competition (iGEM)” which moved from Boston (MIT) to Paris in 2022.
Main challenges and objectives
Researchers from the communities of synthetic biology, biotherapy and bioproduction share the manipulation of living organisms, whose complexity is the root of several scientific and technological challenges. It is therefore necessary to develop approaches that allow production yields that are efficient, standardizable, automatable and compatible with scaling up. It is also imperative to develop innovative, standardizable and high-throughput analytical methods.
BioConvS Aims to synergize different disciplines of life sciences (biotechnology, analytical biochemistry, pharmacology, toxicology, etc.), engineering, modeling or artificial intelligence around different strategic axes related to biosynthesis and biotherapy/bioproduction through 5 main R&D axis.
What is a DIM?
In order to support research development on its territory, the Ile-de-France region focuses its funding on thematics which are considered of general interest and around which a network of universities, institutions, research labs and companis is gathered. This is what we call a Major Research and Innovation Domain (Domaines de recherche et d’innovation majeur, or DIM for short).
BioconvS is part of the 9 DIMs certified by the Région Ile-de-France for 2022-2026, and is carried by the Faculty of Science of the Université Paris Cité.
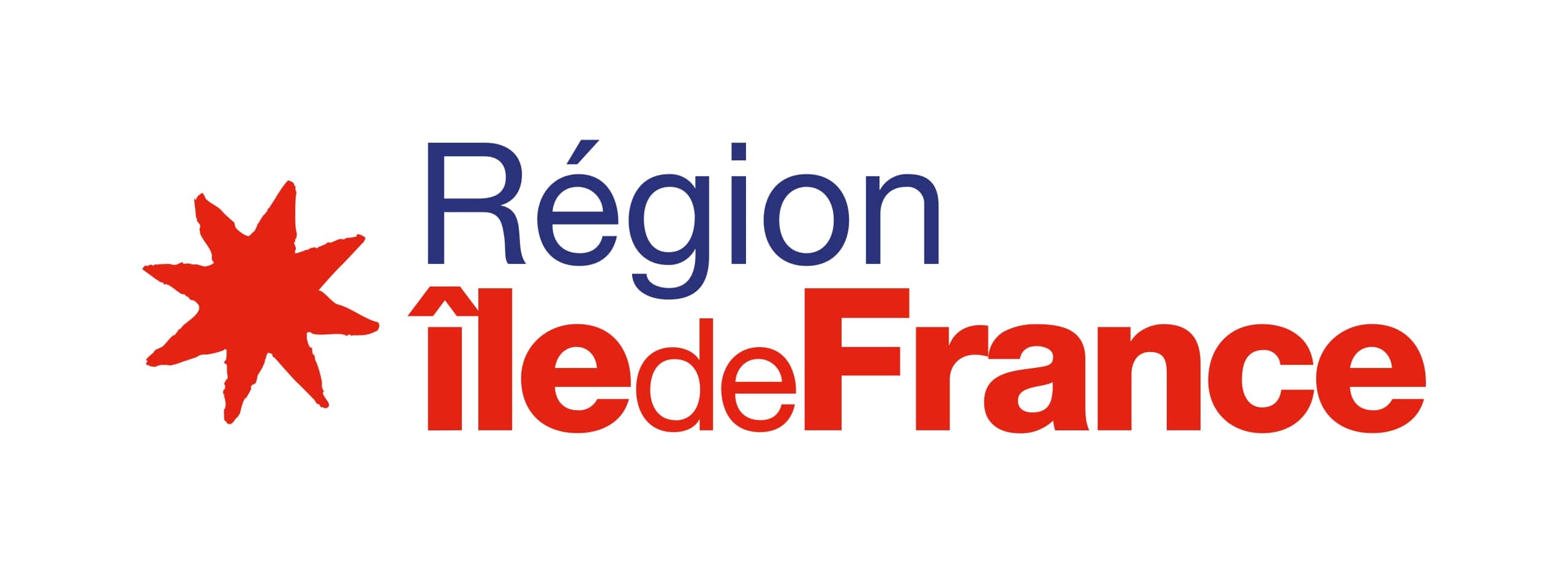
Thematics of the DIM BioConvS
Are you a researcher working in Île-de-France in one of the following thematics or axes? Then you are part of our network and eligible to apply to our calls and initiatives.
Axis 1: Bioengineering and bioproduction
Axis 1 focuses on processes for bioengineering and bioproduction by strengthening the links and possible synergies between them.
With regard to the engineering of life, the key points will be the manipulation of DNA, genetic circuits and cellular processes. Current DNA manipulation methodologies (editing, synthesis and assembly of genes and genomes) can still be improved. They are based on the enzymatic assembly of relatively small fragments (less than 3000 bases), with a fairly low overall error-free yield. However, DNA engineering, the basic material of many biotechnologies, is a key and critical step for many applications. Thus the development of more effective methodologies, more fast and more precise would have an enormous impact both on the level of fundamental research and on companies in the sector. Without claiming to be exhaustive, the following strategies will be investigated: DNA synthesis, DNA assembly and precision editing of the genome (CRISPR).
This axis is also interested in the characterization, production, temporal control, evolution of biomolecules and their assemblies to form circuits, metabolic networks, and predictable, complex, integrated and robust cellular functions. Indeed, macromolecules (nucleic acids and proteins) and metabolites are at the heart of biological functions, driven by evolution to enable extraordinarily sophisticated natural functions, which go far beyond our engineering capabilities. Beyond these molecules, it is our ability to predictively and automatically build cellular regulatory pathways, even chromosomes and artificial cells, that must be significantly developed. Being able to predict, design, generate, assemble, manipulate and control biomolecules and circuits in a way that rivals the complexity and functional richness of cells is the ultimate goal of life engineering. For this, we will discuss in particular the following approaches:
(i) collection, conservation, standardization and reuse of molecular bricks;
(ii) de novo design and on-demand synthesis of macromolecules;
(iii) directed evolution for macromolecular engineering;
(iv) unnatural nucleotide and amino acid polymerization systems;
(v) integrated design of genetic systems for cellular control, information processing and bioproduction.
This axis also includes the domestication, manipulation and use of unicellular hosts, microbial consortia or multicellular organisms. Recent achievements in synthetic biology have demonstrated the ability to modify microorganisms, plants and animal cell lines to perform tasks that nature did not select. In addition to the use of single-celled hosts, the engineering of multicellular consortia, in which multiple cells share specific functions (division of labour), is an effervescent line of research that deserves our attention. Similarly, advances in cell-free systems for the production of macromolecules at the gram/liter scale make it possible to envisage on-demand industrial biomanufacturing applications. The DIM BioConvS will notably support the development of methods, tools and models for:
(i) design synthetic cells and cell-free systems;
(ii) allow for the transformation, modification and reprogramming of new chassis;
(iii) fabrication of multicellular organisms.
Finally, this axis is also dedicated to bioproduction processes for biotherapies. It turns out that biotherapies can be altered in unpredictable ways during the manufacturing process, which can lead to a change in biological activity or the induction of a toxic effect. Biotherapies are very sensitive to environmental factors such as changes in temperature, pH, etc. and also to contamination by viruses, bacteria, bacterial endotoxins, fungi, and mycoplasma from the producing cells, the raw materials used or the manufacturing process itself. The implementation of bioprocesses must take these constraints into consideration, hence the importance of automation via bioreactors which will allow the control of the environmental conditions of the cell culture, their traceability, the reduction of contamination during the manufacturing stages, and to facilitate the scaling up of the process. These efforts are particularly useful in the context of the scale-up necessary for the use of viral vectors for gene therapy. Recombinant adeno-associated viral vectors (rAAV) which are administered systemically require doses of up to 1014 particles per kg of body weight, to obtain the therapeutic effect in the case of the treatment of myopathies. In the context of “live biotherapeutics product” (LBP), i.e. microorganisms derived from the intestinal microbiota used as medicine (new generation probiotics), the production capacity at an industrial level is currently a major obstacle to the development of this approach. These microorganisms require demanding culture conditions, often anaerobic, and which are specific to each microorganism. In this context, the challenge is to set up, in possible collaboration with industrialists, automated processes directly in bioreactors compatible with good manufacturing practices (GMP) in compliance with regulations and adaptable to scaling up. The challenge is also to produce the biotherapies of tomorrow at high yield and at lower cost in a reproducible manner with the pharmaceutical quality required to ensure their efficacy and safety.
Axis 2: Development of therapeutic proofs of principle
The therapeutic activity of biotherapies and synthetic biology approaches for health must be validated through in vitro, ex vivo tests or in appropriate in vivo animal models. This investigation is based on a precise understanding of the pathological mechanisms. It is therefore useful to rely on cells by creating in vitro models of diseases by genome editing, for example. On the in vivo level, models induced pharmacologically, by surgical or genetic intervention (in which a gene of interest has been rendered inoperative – knock-out) represent tools of interest for pathological modeling.
This axis focuses on the development of therapeutic proofs of principle for biotherapies and synthetic biology approaches on different therapeutic indications:
- Combat existing and emerging infectious diseases. The COVID-19 pandemic has highlighted the crucial importance of monitoring infectious agents as well as the need to develop a biological arsenal to combat them. Synthetic biology and artificial intelligence are two essential weapons in this fight.
- Building high-performance diagnostic tools. Develop rapid and inexpensive diagnostic tools. These can in particular be based on proteins (antibodies) or nucleic acids (aptamers) that are easy to produce and store.
- Imagine biotherapies and synthetic biology strategies against microbial infections in view of the threat of antibiotic resistance. It is a public health priority. The engineering of synthetic screening systems, the identification of new bioactive natural products, targeted phage-based therapies or microbiome engineering are promising avenues.
- Think about the methods of fighting the viruses of tomorrow. For example, developing new vaccination strategies to achieve animal and human vaccination at very low cost, based in particular on the modular engineering of antigens and adjuvants, using new models (plants, cell cultures, systems without cells) to produce vaccines, exploit microbe immune systems as antiviral strategies.
- Treat chronic and non-infectious diseases.
- Design biosensors to measure metabolites, proteins and other biomolecules in vivo
- Develop the use of bacteria for therapeutic purposes.
- Advance the engineering of cellular consortia (including the human microbiome and the immune system).
- Integrating living cells into “smart” tissues to detect and act preventively (e.g. antimicrobials).
- Develop bioproduction and biotherapy approaches concerning gene, (sub-)cellular or microbiota-based therapy to treat chronic and non-infectious diseases
- Fight tumors
- Develop bioproduction approaches concerning the adoptive transfer of T-CAR cells as well as therapeutic and pre-clinical proof of principle for these biotherapies
- Develop bioproduction approaches regarding subcellular or microbiota-based therapy and their evidence of therapeutic efficacy for anti-tumor therapy
- Treat rare diseases
- Design new gene correction technologies via viral vectors from lentiviruses, adeno-associated viruses, etc. for rare diseases
- Develop bioproduction approaches for (sub-)cellular or microbiota-based therapy
- Develop therapeutic and pre-clinical proof of principle for biotherapies concerning rare metabolic and immune system diseases, myopathies, etc.
From a clinical translation perspective, the aim is also to use therapeutic efficacy tests capable of predicting whether a batch has the potential to achieve the expected therapeutic effects in humans. These are “potency” tests, with a validated methodology and determined acceptance criteria. These in vitro and/or ex vivo tests may constitute indicators of normal or pathological biological processes capable of responding to the action of the therapy. In some cases, the target of the “potency” test may subsequently be considered as a biomarker during clinical trials.
Axis 3: New, high throughput and standardizable analytical methods
Analytical methods are at the heart of the implementation of synthetic biology approaches and bioprocesses for the production of biotherapy. Analytical methods highlight the impact of input variables (operating parameters such as culture conditions, raw materials) on the output data obtained (performance and quality attributes). A major challenge will be to set up new quantitative methods from biology, physics, chemistry, bioinformatics used for high-throughput characterization within the framework of synthetic biology approaches and biotherapy.
From a clinical translation perspective, analytical methods are of paramount importance in defining optimal operating ranges for bioprocess parameters, acceptance criteria and, therefore, product specifications in compliance with regulations. In this context, the challenge is to develop, in possible collaboration with industrialists, analytical methods dedicated to the specificities of each type of biotherapy, to adapt consolidated methods for a type of biotherapy to another, to standardize these methods and carry out their analytical validation (e.g. determination of specificity, repeatability, reproducibility, linearity, detection limits, quantification limits) according to the current regulatory requirements. The challenge is also to set up in-line controls with the least impact on yield, making it possible to stop a costly process as soon as possible when it does not meet the essential batch validation criteria.
Axis 4: Digital or AI tools to improve data analysis or modeling in quality control and production methods
Despite rapid development and the emergence of new modeling, learning and automation tools, current approaches are still limited by the intrinsic difficulty of predicting quantitative phenotypes and controlling their behavior, especially when scaling up from benchtop (small scale) experiments to medium and large scale production. We will focus on artificial intelligence approaches for model inference, experimental planning and sampling.
- Improve bioengineering prediction and design algorithms at the molecular, cellular and multi-cellular scale. Computer tools facilitating the design of proteins and functional oligonucleotides have benefited from many advances in recent years but remain limited to a few functional classes (transcription factors, enzymes, etc.). There is a need to develop more generic design algorithms based on experimental data, artificial intelligence, and principles of physics and chemistry. The DIM BioConvS will support the extension of computational models by incorporating other data (e.g. multi-omics data, physico-chemical environment).
- A computational infrastructure in support of the DBTL cycle. Many computer tools have been developed in recent years to cover all stages of the DBTL cycle. However, these tools remain difficult to use (they are not necessarily open-source or require specific expertise) and cannot be interfaced (inputs/outputs are not always standardized). Some biofoundries have developed their own tool chains but these are not freely available and are specific to the instruments and methods used by these biofoundries. Ideally, a synthetic biology informatics infrastructure should be generic, facilitating the addition of new software, and should make a set of informatics tools easy to find, accessible (open access), interoperable and reusable, in accordance with the principles FAIR promoted by Europe and France in the conduct of research projects. BioConvS has chosen to use the Galaxy-SynBioCAD platform which makes it possible to meet the constraints set out above. The platform is installed on the Galaxy workflow system widely used by several communities in the life sciences (bioinformatics, genomics, metabolomics, proteomics, cancer, plants, drug-design) and chosen by the European ELIXIR infrastructure. We will complete these tools with the development of new machine learning methods and the control of robotic workstations in our biofoundry.
- IT tools for setting up bioprocesses and quality control. Considering the power of current computer tools, their use should be more and more widespread to help set up bioproduction processes and develop innovative analytical methods. For example, the use of numerical simulation of fluid mechanics is one of these tools for simulating flows in bioreactors. Computer tools, in particular artificial intelligence, are also interesting for the development of innovative analytical methods with high information content. Artificial intelligence makes it possible to process a large amount of multimodal data, select the modalities, reduce them to the relevant data, express probabilities via projections or predictions that can go as far as prescriptive actions, assets that could be exploited for quality control biotherapies. In this context, the interdisciplinary challenge, in collaboration with mathematicians, physicists, computer scientists and any other discipline outside the field of life sciences, will consist in improving the performance of bioprocesses and analytical methods, in order to better control bioproduction and quality control of tomorrow’s biotherapies, in possible partnership with manufacturers.
Axis 5: Ensure a responsible, ethical and inclusive Bioconvergence
It is essential to include in the scientific program of the DIM the ethical aspects associated with the uses of living organisms for medical, societal and/or economic progress. In particular, and without being exhaustive, we will encourage projects taking into account the following elements:
- Risk and biosafety studies, including new methods of risk assessment, the engineering of synthetic safety systems, and the responsible dissemination of synthetic biology tools among the public, students, and hobbyists (i.e. called “garage biology”).
- Study of the ethics of engineering living systems and their manipulation during bioproduction.
- Support open communication between DIM researchers and public actors who could be impacted by this research and the deployment of new technologies. This will include studies on how to set up a co-design process to translate societal challenges into open research projects (citizen/participatory science).
- Learning from history by understanding the processes that led to a science-society divide in order to inclusively share biology-engineered innovation.
- Understand how potential resolutions of sustainability challenges through bio-engineering, -production – therapy translate into practice, new policies and ethical guidelines
- Integrate social studies with bioengineering, bioproduction and biotherapy from the start of the project, leading to co-publications and developing and valuing different philosophical positions
- Develop approaches that direct bioengineering and biotherapies towards reducing inequalities and increasing well-being to support its impact on innovation, the engine of economic growth.
Our topics of interest
- Cell therapy
- Gene therapy
- Viral vectors
- Vaccines
- Bioengineering approaches to tackle non-communicable diseases
- Bioengineering approaches to tackle cancer
- Bioengineering approaches to tackle rare diseases
- Synthetic biology
- DNA manipulation
- Synthetic gene circuits
- Synthetic metabolic networks
- Standardized biobricks
- Gene Editing techniques
- Extracellular vesicles
- Bioproduction
- Acellular systems
- Automation of bioprocesses
- Directed evolution
- Non-natural aminoacids
- Synthetic cells and organisms
- Microbiota
- New generation probiotics
- Innovative diagnostic systems
- Antibiotics resistance
- Biosensors
- Cellular consortia
- Innovative quantitative analytical methods
- Computational approaches for model inference
- Computational approaches for experimental design
- Computational approaches for experimental analysis
- Computational tools for bioproduction and/or quality control
- Bioethics